The Hammond research group focuses on the self-assembly of polymeric nanomaterials, with a major emphasis on the use of electrostatics and other complementary interactions to generate functional materials with highly controlled architecture. The uniting theme of the lab – the understanding and use of secondary interactions to guide materials assembly at surfaces and in solution – encompasses four major areas of research:
1) Layer-by-layer controlled release thin film coatings for biomedical implants that address bone regeneration, wound healing, tissue engineering and transdermal delivery from microneedle platforms,
2) Nanoparticle drug carriers for targeted nanoparticle drug, gene, and siRNA delivery for cancer treatment using unique platforms that enable combination and sequenced delivery strategies,
3) High-throughput, data-driven layered nanoparticle screening and design,
4) Engineering synthetic blood substitutes using polymer-based hemoglobin carriers and artificial hemostat for improved oxygen transport and coagulation control.
Charge-based Assembled Biomaterials for Regenerative Medicine
Our lab has developed approaches around the incorporation of surface-erodible, hydrolytically degradable components in coatings for sequential or simultaneous multi-drug release. These advances led to the controlled release of proteins and biologic factors from conformal coatings of implants and scaffolds that yield physiologically relevant amounts delivered locally over extended periods of up to multiple weeks. Very recent results from our research group indicate the success of delivery of bFGF, BMP-2, VEGF and PDGF, as well as nucleic acids such as plasmid DNA and siRNA. We have applied electrostatic layer-by-layer and other polymer self-assembly processes toward biomaterials for applications including tissue engineering and wound healing, including diabetic ulcer wound healing, soft tissue wounds and antifibrotic healing processes. By directly incorporating siRNA to knock down specific proteases, we have also shown the promise of nucleic acid delivery directly to wound sites, and the use of DNA as a vaccine delivered with microneedle technology.
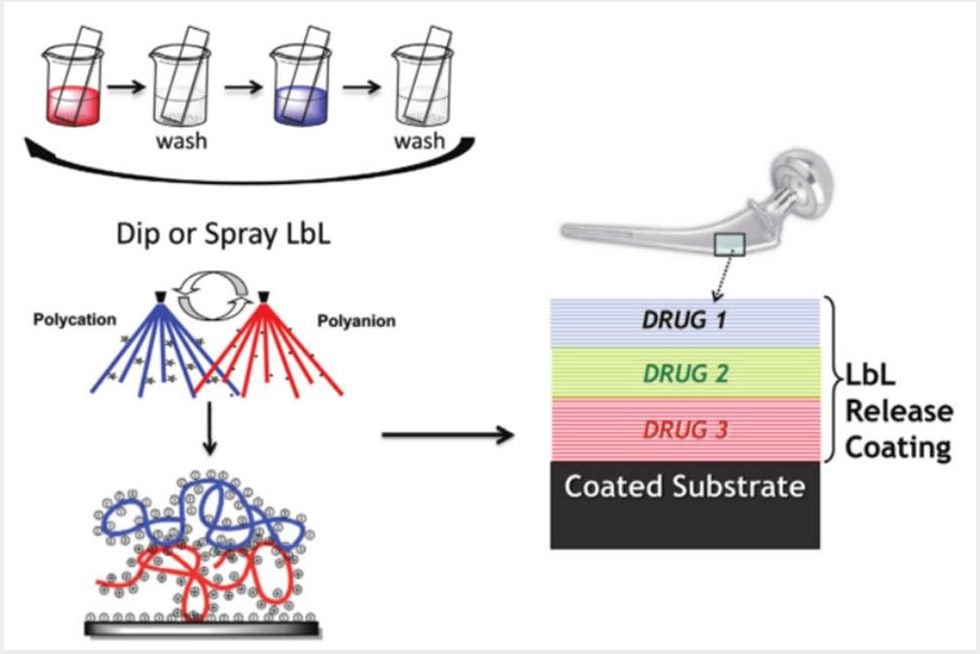
Targeted Nanoparticle Delivery
By adapting the methods of layer-by-layer (LbL) from large surfaces to nanoparticles, it is possible to gain many of the same advantages described above toward the design of nanoparticles that can target cancer based on size and molecular targeting, while enabling the release of multiple agents optimized to achieve maximum impact and synergistic cancer cell death. The Hammond lab has applied these LbL concepts to nanoparticles to address the targeting of cancer. The nanoparticle systems can be tuned to release an agent such as an inhibitor or siRNA that blocks the defense mechanisms of tumors, followed by delivery of a chemotherapy drug that can then exhibit enhanced efficacy. Additional approaches include micellar assemblies with siRNA and ligand-cluster dendritic block copolymers that provide a means of enhanced targeting. The lab has recently investigated concatenated siRNA approaches using rolling circle transcription toward the design of self-generated porous polymeric RNA microcarriers, which break down into short siRNA strands once within the targeted cells, to yield highly potent and concentrated doses of siRNA.
High-Throughput, Data-Driven Layered Nanoparticle Screening and Design
Our lab has demonstrated that rational design of particle surface chemistries, using polymeric outer layers, yields targeting advantages. To better understand the rules governing these behaviors, libraries of layered nanoparticles, varying core and surface chemistry, have been screened across a broad array of DNA-barcoded cancer cell lines. ML-driven analysis of the data generated has illustrated that NP cores govern cellular uptake as well as surface chemistries; additionally through correlative genomic analysis, we identified key cancer biomarkers that dictate NP trafficking for certain core chemistries. Future work in this area will expand this analysis to broader nanoparticle and cellular libraries, in addition to integrating additional computational techniques to facilitate nanoparticle design.
Polymeric Hemoglobin Carriers and Artificial Hemostats for Improved Oxygen Transport and Coagulation
By leveraging biomaterials chemistry, we aim to design innovative approaches for controlling hemostasis, enhancing wound healing, and developing artificial oxygen-carrier. Our lab has developed interactive two-component systems, consisting of targeting and crosslinking components, for the treatment of internal bleeding by amplifying platelet recruitment and mitigating plasminolysis for greater clot stability. This work addresses the need for supplementation of the native hemostasis process and provides guiding principles for future development of artificial blood replacement. To improve translatability of our technology, we are further investigating hemoglobin-based oxygen carriers amenable to freeze-drying and long-term storage.